In this article, we will explore how quantum computing is transitioning from a complex theory to a reality with practical applications for an increasing number of companies.
Quantum computing represents a revolutionary breakthrough in technology, offering an entirely new way of processing information.
Unlike classical computing, which uses bits to represent binary values of 0 or 1, quantum computing relies on qubits, which can exist in multiple states simultaneously thanks to superposition.
This principle, combined with quantum entanglement, allows calculations to be performed at a speed and scale unattainable by traditional computers.
Interest in quantum computing has grown exponentially in recent years. The awarding of the 2022 Nobel Prize in Physics to Alain Aspect, John F. Clauser, and Anton Zeilinger for their research in quantum information highlights its transformative and revolutionary role.
This emerging technology, which we already use at VASS, has the potential to transform entire sectors, from logistics to finance, optimizing complex processes and solving problems that were previously unapproachable.
However, the path to fully functional quantum computing presents significant challenges. Reducing noise and improving quantum coherence are key areas of research. As progress is made in these areas, the practical applications of quantum computing will continue to expand, opening new possibilities for innovation and technological development.
In this article, we will explore how quantum computing is transitioning from a complex theory to a reality with practical applications for an increasing number of companies.
What is Quantum Computing?
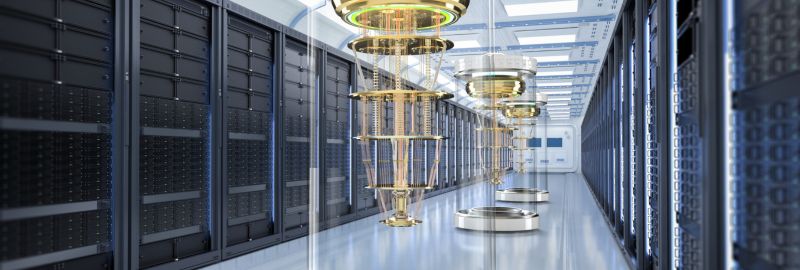
Quantum computing is a computational paradigm based on the principles of quantum mechanics, a branch of physics that studies the behaviour of particles at the subatomic scale.
In contrast to traditional computing, which uses bits to process information as values of 0 or 1, quantum computing employs qubits. A qubit can simultaneously represent both states, 0 and 1, thanks to a phenomenon known as superposition.
In addition to superposition, another key concept in quantum computing is entanglement. When two qubits are entangled, the state of one instantly depends on the state of the other, regardless of the distance between them. This phenomenon enables the creation of highly interconnected systems with exponentially greater processing capacity than classical computers.
Through these principles, quantum computing can solve problems that would be practically impossible to tackle with traditional methods. However, its development is still in an early stage, and we are only beginning to glimpse its potential.
Quantum noise management and qubit stability still prevent this technology from reaching its full potential.
Fundamentals of Quantum Computing
To better understand quantum computing, it is essential to review the fundamental principles of quantum mechanics and the description this branch of physics provides of particles at the subatomic level. As mentioned earlier, the two key concepts that make quantum computing possible are superposition and entanglement.
Superposition
In classical computing, a bit can be in one of two states: 0 or 1. However, a qubit, the basic unit of quantum computing, can be in a combination of both states simultaneously. This is known as superposition.
It is a concept that is difficult to grasp. To illustrate, we can imagine a qubit as a coin spinning in the air; while spinning, it is neither heads nor tails but a mixture of both. Thanks to superposition, a quantum computer can process multiple possibilities simultaneously, exponentially increasing its computational capacity.
Entanglement
Quantum entanglement is a phenomenon where two or more qubits become interdependent, so that the state of one instantly affects the state of the other, regardless of the distance between them.
Continuing with our previous analogy, it is as if two spinning coins were synchronized in such a way that when one lands on heads, the other does as well at the same time, no matter how far apart they are.
This phenomenon allows quantum computers to perform calculations extremely efficiently, as they can coordinate information between qubits in a way that has no equivalent in classical computing.
Types of Quantum Computers
However, not all quantum computers are the same. In fact, there are different approaches to building quantum computers, with the two most important being:
- NISQ (Noisy Intermediate-Scale Quantum) Computers: Based on quantum circuits, they use logic gates to manipulate the states of qubits. These gates perform quantum operations such as rotations and entanglements to execute algorithms.
- Adiabatic Quantum Computers: These machines are designed to find solutions to complex problems by searching for the minimum energy state of a wave function. They are particularly useful for solving optimization problems.
Differences Between Quantum Computing and Classical Computing
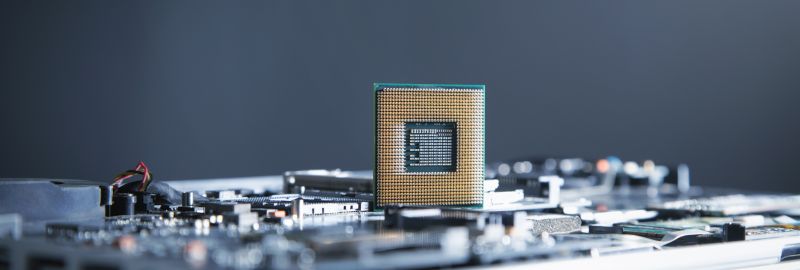
Quantum computing and classical computing are profoundly different paradigms, both in their operation and their capacity to solve problems.
Here are the main differences between the two:
Unit of Information
As we have emphasized, because it is the foundation of everything, in classical computing, the basic unit of information is the bit, which can be either 0 or 1, meaning it has only two states.
In contrast, in quantum computing, the basic unit is the qubit, which can represent 0, 1, or both simultaneously due to superposition. In other words, it has three states, allowing quantum computers to perform more tasks at once.
Computational Power
Classical computing processes information linearly, with processing speed directly dependent on the amount of available resources. In other words, calculations are performed sequentially, one after the other.
Quantum computing, however, exhibits exponential growth in computational power due to qubits' ability to handle multiple states simultaneously. This allows quantum computers to perform multiple calculations at once, reaching conclusions much faster than classical methods.
Noise Management
Classical computing operates in a noise-free environment, meaning bits maintain a stable state during processing, resulting in virtually error-free operations.
Quantum computing, however, is inherently noisy. Qubits are sensitive to their environment, which can affect their stability and cause errors, a phenomenon known as "quantum noise." This is currently the main challenge in quantum computing, although efforts are being made to harness this noise.
Currently, research is being conducted to develop methods for leveraging this noise.
Types of Applications
Classical computing is well-suited to efficiently solving a wide range of problems but encounters limitations with problems requiring exponential computation. Everything we do today with a classical computer uses this type of computing.
On the other hand, quantum computing is especially efficient for certain types of problems, such as large number factorization, optimization, and molecular simulations, where it far outperforms classical computing.
Algorithms and Logic Gates
Classical algorithms are designed to operate with bits and traditional logic gates (AND, OR, NOT). We are all accustomed to using these in our everyday computing tasks.
In quantum computing, however, algorithms are built on quantum logic gates that manipulate qubits and exploit phenomena such as superposition and entanglement. This allows quantum computing to perform tasks that classical computing simply cannot handle.
In summary, quantum computing does not replace classical computing but rather complements it for specific applications. While classical computers will continue to be useful for many everyday tasks, quantum computing opens new frontiers for problems that are currently unsolvable with conventional technology.
Practical Applications of Quantum Computing
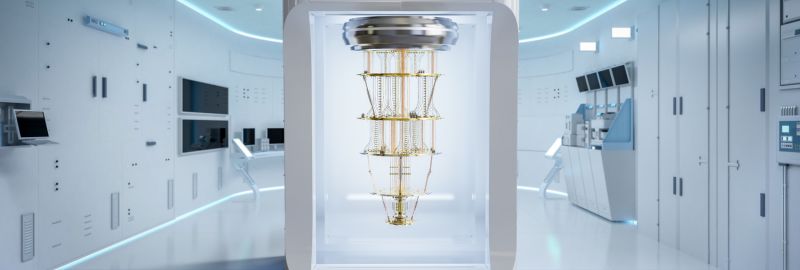
Quantum computing is already demonstrating enormous potential in various areas, many of which we work on at VASS. Thanks to its ability to process information exponentially faster than classical computing, multiple practical applications in key sectors are being explored. Here are some of the most important that are already in practice:
Quantum Computing in Logistics
Reservoir Management: In the gas industry, it is crucial to maintain optimal levels in reservoirs to meet demand without incurring high costs. Quantum computing can optimize the use of pumps to manage gas flow, minimizing operational costs.
Route Planning: Optimizing transportation routes considering variables such as distances and gradients is a complex problem. Quantum algorithms can find the optimal combination of transportation modes to maximize efficiency and minimize costs.
3D Container Packing: In the transportation and logistics sector, quantum computing can help solve the problem of three-dimensional packing, using the minimum number of containers possible for items of different sizes and shapes.
Quantum Computing in Finance
Investment Portfolio Optimization: Quantum computing can enhance the selection of financial assets that maximize returns and minimize risk, solving complex optimization problems that are challenging for classical methods.
Option Pricing: Pricing financial options is a computationally intensive task. Quantum algorithms can accelerate these calculations, improving both accuracy and efficiency.
Arbitrage: Quantum computing can identify arbitrage opportunities—where profits are made from price differences between similar financial instruments—more quickly and accurately.
Credit and Market Risk Analysis: Quantum models can more rapidly assess the probability of defaults or market fluctuations, helping financial institutions make more informed decisions.
Applications in Simulations and Science
Simulation of Complex Systems: In fields such as chemistry and materials science, simulating molecules and reactions is vital but extremely complex. Quantum computing can perform simulations that were previously impossible, accelerating the development of new materials and drugs.
Quantum Monte Carlo: Monte Carlo simulations are techniques used to model probabilities in complex processes. With quantum computing, these simulations can be performed much faster, benefiting sectors such as particle physics and climatology.
Artificial Intelligence and Machine Learning
Quantum Machine Learning (QML): The integration of quantum algorithms in machine learning promises to improve tasks such as fraud detection, recommendation engine optimization, and the classification of large data sets. In particular, quantum computing can make AI models faster and more efficient.
Although the applications of quantum computing are still in an early stage, progress is rapid and already transforming multiple industries. From logistics to finance, and from science to artificial intelligence, the ability to solve complex problems efficiently opens new possibilities that will revolutionize technology and the global economy in the coming years. Only the companies that start working with it today will be able to lead tomorrow.